We are atom
coolers in Aephraim
Steinberg's research group. We trap and Cool Rubidium 85 atoms
using the
usual MOT-molasses method in a vapor cell and
perform various experiments on them. The other experimentors on
these
projects are graduate student Samansa Maneshi and our post-doc Matt
Partlow.
|
|
We
have other experiments and other grad
students in the lab, but I try
not to let that bother
me. They are:
Jeff Lundeen
Mirco Siercke
Chris Ellenor
Rob Adamson
Krister Shalm
Fan Wang
The Quantum Delta Kicked Rotor with Cold Atoms
The
classical delta-kicked rotor (DKR) exhibits chaotic behaviour in
certain parameter regimes, characterized by a linear increase of the
energy of the system as a function of time. Applying the DKR to a
quantum system, however, will eventually result in states that are
localized in momentum space. Therefore, the delta kicks can only
increase the energy of the system up to a finite limit given by the
momentum localization length. Recent extensions of the quantum DKR by
P. Brumer and J.Gong
predict coherent control of the relaxation of the system into the
localized eigenstates through manipulation of the coherence properties
of the initial state and spatial phase of the pulsed potential. This
can result in either a further suppression of diffusion or superheating
in which the system absorbs energy faster than classically allowed
|
|
Quantum Error Correction
|
Our
optical lattice can be tailored to support only two bound states. Phase
modulation of the lattice can create various
superpositions of the two bound states, known in the quantum
information world as a qubit. Because of imperfections in the
sinusoidal potential these qubits
undergo dephasing and decoherence. Further phase (and/or intensity)
modulations can correct for these dephasing mechanisms. We are studying
techniques to preserve the coherence of our qubits not just in the face
of our dephasing mechanisms, but to realize a generalized technique
through optimization algorithms to find correction schemes for a range
of dephasing/decoherence mechanisms.
|
Quantum Process Tomography of an Optical Lattice System
By
subjecting four known independant states to an unknown time-dependant
potential and subsequently measuring the output density matirces one
can construct the SuperOperator of the potential. This operator fully
characterizes the system and predicts the output state for any given
input state. We reconstruct this superoperator for various processes in
an optical lattice. Processes measured include resonant shaking of the
lattice, instantaneous shifts of the lattice and what should be the
Identity (doing nothing to the lattice) but isn't because of
dephasing/decoherence mechanisms.
|
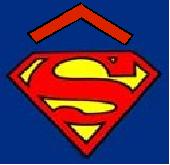 |
Quantum Tomography of Atoms in an Optical Lattice
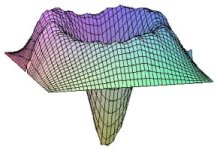 |
The
individual wells of a 1-D optical lattice
can be approximated by a
harmonic potential. We have mapped the motional density
operator
of atoms in this potential in the number state basis. The same theory
for
tomography for photonic states is applicable here. What we actually
measure is the
quasi-probability distribution known as the Husimi, or Q, distribution.
It
is always real and positive, thus measurable in the laboratory.
However,
it still
has all the information as the Wigner distribution or the density
operator. |
Velocity Selection of Atoms in a Magnetic Trap
Atoms
are trapped
in a weak quadrupole magnetic trap, and a blue-detuned laser sheet
is placed to the side of the trap. The magnetic trap is then moved past
the laser sheet. The repulsive potential due to the blue-detuned laser
prevents the colder atoms (those with less kinetic energy than the
laser
potential) from moving past it, separating them from the more
energetic atoms which can classically
overcome this potential. The result is a cold sample of atoms spatially
separated
from the original atom cloud. |
|
Magnetic Field Delta Kick Cooling
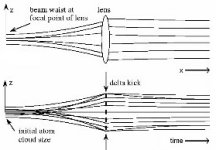 |
By
application of a pulsed magnetic field,
laser-cooled Rb atoms are
cooled in one dimension, by a factor of 10 below the
temperature of
optical molasses. While phase-space density is conserved in this
process,
the method is more efficient than adiabatic expansion. We have achieved
temperatures below 700 nK, but there is no fundamental limit to this
cooling technique. The technique can also be used as an atom lens and
as a
spin-dependent probe.
|